Principles of Zener Barriers
A Zener barrier is an associated equipment that
is installed in the safe area. It is designed to limit the amount
of energy that could appear in an electrical circuit passes through
the hazardous area despite the connection before the barrier.
A barrier consists of:
Resistors to limit
the current
Zener diodes to
limit the voltage
Fuses to protect
the components
As any intrinsic safety equipment, the Zener barrier allows cables
to short circuit to each other or to metallic parts connected
to ground without danger.
The Zener barrier interfacing mode differs from others as there
is no galvanic isolation. Cables that pass through the hazardous
area thus share common features with those of the safe area.
This implies equipotential grounding.
Figure (1) illustrates an intrinsic safety equipment (A) connected
to a circuit (C) through a Zener barrier (B) that limits the
current, the voltage and the power.
If a fault voltage occurs between the terminals (m) and (n),
the Zener diode (protected by a fuse) limits the voltage that
risks appearing in the hazardous area and the resistor limits
the current to an acceptable value.
If a fault voltage occurs between the terminal (m) or (n) and
the ground, the voltage of the wires (e) and (f) relative to
ground will not exceed Vz provided that the Zener barrier is
correctly grounded at (T1).

Current path in normal operation, U <= 9V
Current path in the overvoltage case, U <= 9V
The Zener diode becomes conducting
The fuse protects the Zener diode from destruction
The Zener barrier permits wires (e) and (f) to be short circuited
without danger. However, if point (n) accidentally reaches a
high potential relative to ground, a ground fault at (f) risks
causing a dangerous spark.
To ensure the safety of such a wiring, point T1 must be connected
to ground as illustrated in Fig.(2). So, in the event of a fault
between (m) and (n), the voltage between (e) and (f) will not
exceed Vz, the short circuit current between (e) and (f) will
not exceed Vz/R, and the ground fault current will be zero for
point (f), and equal to Vz/R for point (e).
Notice:
To validate the following statement, grounds T1 and T2 must be
at the same potential.
Actually, a Vt difference of potential causes a loop current
only limited by line and ground resistances.
Conclusion: Only an equipotential ground network can ensure the
safety of a reference ground system.

A wide range of barriers has been developed to fit all type of
installation. They differentiate by their electrical circuit
diagrams, parameters and functions.
The electrical circuit diagram differs from a barrier to another.
There are
two main types:
The 'single' barriers: The 'double' barriers:

Single
barriers:
In this configuration, one of the two metrologic wires is directly
connected to the ground (T1) at the barrier (Fig. (2)).
If there is a difference in the potential between T1 and T2,
a ground fault (T2) can cause a loop current to occur.
Even if this current does not affect the system, it can impair
the measurement of low level signals (e.g. Pt100, thermocouple).
An important difference of potential can degrade the safety.
Double
barriers:

Vt
= Difference of potential between grounds T1 and T2
With a barrier of this type (Fig.(3)), a ground fault at (f)
causes a loop current to occur:

The current It is lower than with a single barrier where it can
take the value:

Another advantage is that the double barrier - unlike the single
- ensures isolation of the metrologic wires relative to the ground
corresponding to the Zener diode threshold.
The barriers
and their functions
Three main functions:
A signal current
transmission function
A signal voltage
transmission function
A power supply
function
In the current signal transmission function, the measured
value is the current. The barrier is integrated in a loop connected
to a current source. The barrier's protective diodes must not
conduct.
The barrier brings an additional resistance which must not cause
the acceptable loop resistance to be exceeded. The barrier is
determined as follows:

Ue is the voltage for which a leakage current lower than or equal
to I(t) is ensured.
If I is the current and V is the voltage needed in the hazardous
area (fig. 4): The following relations must be checked for the
equipment operates correctly:
V + I ×
(Rs + Rc) < Ue
U < Ue
If these relations are checked, the maximum ohmic value of the
cable can be determined as follow:
Rc max. = [Ue
- V - (Rs × I)] / I
The fuse resistance R Fu is so negligible that calculation can
be made with the value RL of the bzg parameters instead of Rs.
Influence
of the Zener barrier internal resistance:
Because of V - the voltage needed for the equipment operates
in hazardous area-, the loop resistance Rc + RL must be compatible
with the supply voltage at the barrier input.
An associated equipment has always an internal resistance (RL)
in series with the terminals connected to the intrinsic safety
equipment.
The intrinsic safety parameters Po and Io of the associated equipment
(See chapter 1.3) are determined by this resistance.
This resistance RL can affect the operation of the connected
intrinsic safety equipment by generating a voltage drop at its
terminals:
^u = (RL + Rc)
x I consumed by the transmitter
Example
:
A 24V supplied transmitter through a Zener barrier with an internal
resistor of 200O. The cable resistance is negligible.
Maximum current
consumed by the transmitter: 21 mA
Voltage drop ?u
due to the internal resistance: 0.021 x 200 =4.2V
Effective supply
voltage V seen by the transmitter: 24-4.2= 19.8
V
In this example, RL is included in the calculation of the maximum
load resistance specified by the transmitter manufacturer.
The effective supply voltage V must never be lower than the minimum
supply voltage specified.
The voltage, current and power values limited by the barrier
must also be considered.
The equipment operating voltage in the hazardous area must be
lower than the Uo of the associated equipment.
The equipment operating current in the hazardous area must be
lower than the Io of the associated equipment.
The operating power consumed by the equipment in the hazardous
area must be lower than the Po of the associated equipment.
The voltage signal transmission function implies to check
that the barrier resistance RL does not badly weaken the signal
when the receiver input impedance Z is not illimited (fig. 5).

The value of the pulse V must be lower than Ue, otherwise the
generator risks short-circuiting through the resistor Rs. The
pulse frequency must be checked.
The power supply function is as follow (Fig. 6):
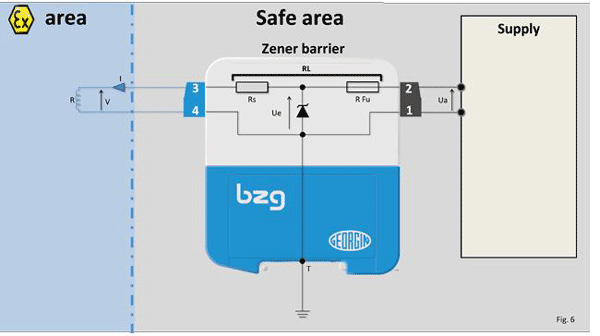
For this type of barrier the following relations must be checked:
If I is the current needed in the hazardous area.
I <= Ue / (Rs
+ R)
The fuse resistance R Fu is so negligible that calculation can
be made with the value RL of the bzg parameters instead of Rs.
This information kindly issued by :
REGULATEURS GEORGIN UK
Newbold Road Rugby CV21 2NH
Email :uk@georgin.com
Tel : 0044(0)1.788.557.413
Web : www.georgin.com
November
2012
|



|